PNAs: An amazing molecular tool that can be converted into an effective antibiotic
One of the most underappreciated aspects of biochemistry is the incredible variety of molecular tools we have available. For example, if I was working with DNA in lab, I could replicate it with a polymerase, cleave it at specific sites with restriction digest enzymes, connect it to another strand of DNA with ligase, and use this DNA to transform cells within a day or two. Alternatively, I could label the DNA with fluorescent nucleotides, hybridize it to fixed cells on a slide, and look at where it localizes under a fluorescent microscope in the same timeframe. These steps might feel routine after I’ve done them 20 times in a row, but each of them involve amazing interactions facilitated by reagents that have been developed and optimized for years until they become standard laboratory tools.
The development of new molecular tools hasn’t slowed down either. New reagents and enzymes are constantly being released, and many researchers devote themselves to improving upon the tools other scientists use. Today, I’m going to focus on one such tool in development and how it went one report on a type of compound with some potential into an entire field devoted to finding new ways to utilize them. These compounds are known as peptide nucleic acids.
What are they
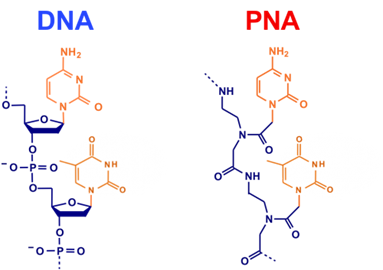
They were first reported in 1991 publication in Science so the discoverers definitely had some idea that this would be a big deal 3. They also reported one of the most important properties of these compounds: PNAs bind very tightly to complimentary DNA via base pairing.
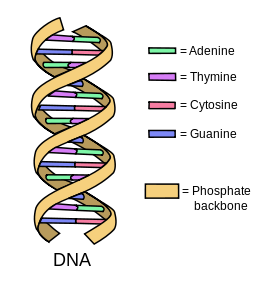
The term complimentary here means that when two strands of nucleic acids line up, each base is paired with the base it normally matches up to on the other strand. Every Adenine (A) will base pair to a thymine (T) while every Cytosine (C) will base pair to a guanine (G) and vice versa. For example, a DNA strand of AAGGGTCCCC will complement TTCCCAGGGG
When two complimentary strands of DNA are aligned like this, the base pairs that form create a relatively strong bond that keeps the DNA strands together and allows them to form the well-known double helix structure.
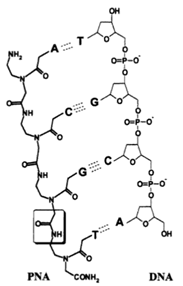
Since PNA is structured in the same way as DNA, complimentary PNA/DNA binding isn’t too surprising. However, the main difference between two DNA strands base pairing and a PNA-DNA interaction is that the connection between PNA and DNA is much stronger. If a DNA double helix was two childhood friends holding hands, a PNA-DNA interaction would be newlyweds on their honeymoon. This is because two strands of DNA both have a negatively charged backbone that are repellant to each other while PNA has no such negative charge to counteract base pairing.
Why are they Important?
That tight bond between complimentary PNA and DNA is the main reason why researchers are so damn interested in PNAs. It’s like a superglue that only binds to a very specific sequence of DNA. Since PNAs can be created with any desired sequence, they can be theoretically used to bind to any stretch of DNA in any organism. This allows any tool developed with PNA to be both powerful and specific.
In addition, this type of compound is entirely artificial. It has not been found in nature and no known organisms can digest or transport it. It’s also quite stable at room temperature. This means that if a PNA was inserted into a cell or a reaction mixture, it would be expected to persist for a long time. Thus, a small amount of PNA can have much longer of an effect than a comparable amount of DNA which is readily digested by most organisms.
Since PNAs have multiple useful properties, researchers have been quick to try to develop them into different tools for a variety of applications. One of the first uses of PNAs was to create fluorescent probes that could bind to specific sequences of DNA. Researchers could use these probes to confirm the presence of a specific gene in an organism (or a specific organism in a culture) and found PNA to be much more effective than DNA because of the tighter binding 6. There are also many reports of using PNAs to treat diseases through targeted gene regulation 7. The next section will go into detail as to how PNAs can be used as antimicrobials, but they can be used to target genetic disorders and cancer as well. I was even able to find some examples of PNAs being used for paper-based assays which is great because I just wrote an article on the topic. In one recent example, PNAs were immobilized on paper to create a detector for human papillomavirus DNA 8.
Alright, now its time to do a deep dive into a PNA paper :) The one I’ve chosen shows how a PNA can be used to kill a specific bacterial pathogen.
Using PNAs as Antimicrobial compounds
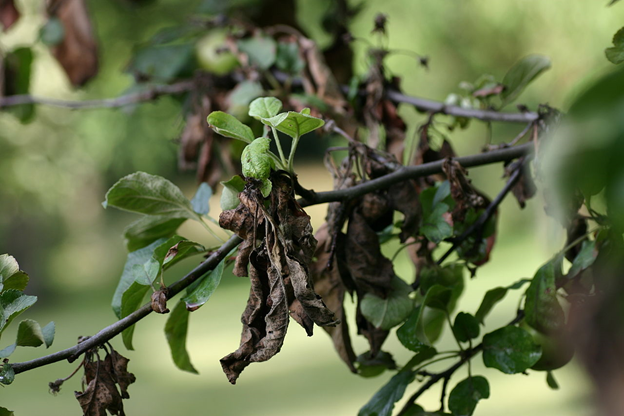
An apple tree infected with Erwinia amylovora showing the symptoms of fire blight 9
Many labs are developing PNAs to combat pathogens and I’ll be discussing a 2017 paper titled “Exploration of Using Antisense Peptide Nucleic Acid (PNA)-cell Penetrating Peptide (CPP) as a Novel Bactericide against Fire Blight Pathogen Erwinia amylovora” to illustrate just how this can be done 10. In this paper, the authors used PNAs to kill a well-known bacterial plant pathogen called Erwinia amylovora. This strain is known to cause blight in apple trees and has a significant agricultural impact. I like this example of developing PNAs into a tool because it it combines PNAs with another major field of research known as RNA silencing to combat a real-world problem. RNA silencing is a big enough field that it would take an entire article to properly explain it, so I’ll have to settle for the overly simplified explanation below:
Scientists have found that if they add RNA complimentary to a gene in a cell, the expression of the gene is prevented by a process known as RNA silencing 11. It turns out that PNA can silence gene expression as well. In fact, since PNA won’t degrade over time and binds very tightly to nucleic acid, a small amount of PNA can be much more effective than a large amount of RNA.
The main issue with using PNAs as gene silencers is that these artificial compounds don’t readily enter cells. Bacteria are surrounded by a membrane that can be very selective in what it allows through and PNAs aren’t on the list. To get around this, the researchers attached a small protein chain called a cell penetrating peptide to the PNA. The cell penetrating peptide is a small protein that hijacks the bacterial membrane’s natural transportation system to trick the cell into importing foreign chemicals 12. The mechanism by which this happens is still debated, but the end result of the PNA getting into the cell is universally agreed to be effective.
Once the PNA is inside the bacteria, the researchers wanted it to effectively kill the organism. Since they could design the PNA sequence to downregulate any gene they wanted, they wanted to prevent the expression of a gene that was essential for the bacteria to survive. They chose to downregulate the gene encoding acyl carrier protein (acpP) which is needed for a critical step in the synthesis of the cell membrane.
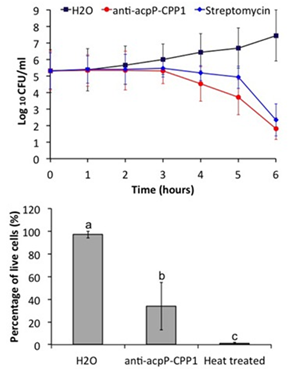
The above experiment is definitive as to the effectiveness of the PNA against Erwinia amylovora, but as with any other research paper, they did a bunch of other experiments backing this result up. They showed that PNA without the cell penetrating peptide failed to kill the bacteria, they showed that the expression of acpP was downregulated by the PNA, and that apple tree flowers infected with the pathogen grew better in the presence of the anti-acpP-CCP compound. The latter experiment was, in my opinion, the most important because it showed that this antimicrobial PNA can actually help protect crops.
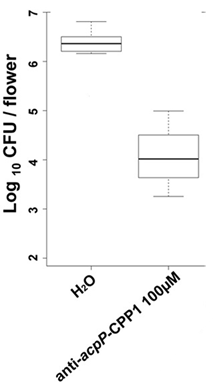
The PNA kills Erwinia on Apple tree flowers (10).
One amazing aspect of using PNAs as antimicrobials is their specificity. Plants have a diverse microbiome and disrupting that with a general antibiotic like streptomycin can hurt the plant. That’s why the authors tailored the PNA sequence to specifically target the acpP gene of Erwinia amylovora. They found that this specific PNA did not affect the growth of most of the other major bacteria that normally reside in the apple tree’s microbiome. It also worth noting that if Erwinia amylovora ever developed a resistance to this particular PNA, scientists can sequence the genome of the resistant strain and develop a new PNA designed to target it.
This paper didn’t dive much into the potential harm these PNA-CCP compounds do to humans, but the risk is quite minimal. The acpP gene is not found in eukaryotes so there is little risk of an anti-acpP-PNA downregulating gene expression in plants or animals. Furthermore, cell penetrating peptides have been shown to be quite safe around humans 13 so there is no concern with using the CCP-conjugate.
Despite these findings, the authors recognize that antimicrobial PNAs are limited by one big factor: price. They note that the “the cost of PNA–CPP is about $1000USD/μMol.” That means a liter of bactericidial PNAs at the 100 μM killing concentration shown by the authors would cost $100,000 and I can’t imagine that single liter would go too far on acres of apple trees. However, as soon as mass production methods are put into place, the cost of creating these molecules should reduce significantly.
References
(1) https://en.wikipedia.org/wiki/Peptide_nucleic_acid
(2) http://sites.bu.edu/biomol/projects/pna/
(3) http://science.sciencemag.org/content/254/5037/1497
(4) https://en.wikipedia.org/wiki/Structure
(5) https://www.nature.com/articles/5201226
(6) http://jcm.asm.org/content/37/9/2760
(7) https://www.sciencedirect.com/science/article/pii/S1471489215000922
(8) https://www.sciencedirect.com/science/article/pii/S0003267016314222
(9) https://en.wikipedia.org/wiki/Fire_blight
(10) https://www.ncbi.nlm.nih.gov/pmc/articles/PMC5395615/
(11) https://www.nobelprize.org/nobel_prizes/medicine/laureates/2006/press.html
(12) https://www.ncbi.nlm.nih.gov/pubmed/17635146
(13) https://www.ncbi.nlm.nih.gov/pmc/articles/PMC4783992/
Images
All images were taken either directly from the publication referenced, is available on Wikimedia commons, or labeled for reuse on Google Images. If any image owner has an issue with this article, please contact me and I will address the issue.
That's such an ingenious approach! I'm sure cost will scale as more people take interest in the project and get working on addressing the cost issues.
Also remember not all bacteria has cell walls! They're mostly an issue in gram (+) bacteria but there are lots of pathogenic species which are gram (-) and have very thin cell walls (E.Coli, Klebsiella etc...)
Great post! I really missed reading your writing during my break!
Thanks! Glad to know someone likes my writing!
I'm sure there are different strategies for penetrating Gram + and Gram - cell walls, and I think the membrane is a concern with both types as well. I'm hoping these do get more and more popular over time so costs scale down.
I hope that at least some people whose expertise is not molecular biology will discover this post and understand that science went well beyond the "ordinary life" with the molecules capable of mimicking the DNA.
I have a strange question. There are some experiments that showed the possibility to inherit the artificial nucleotides. Is it possible to do something similar with the PNA?
(*99% that the enzymes will not be able to continue...)
That's an interesting question. I'm not sure of the answer myself, but I think that an unmodified organism would not be able to incorporate PNA nucleotides into their DNA because their DNA assembly enzymes can't recognize the PNA. However, genetically modifying an organism to produce enzymes designed to insert PNA into DNA strands might be possible. It would be a hell of a challenge at any rate, but work on creating artificial nucleotide is already challenging so who knows.
It would also take adding entire metabolic pathways so that the cells would produce the different PNAs. DNA and RNA differ by a single change of a hydrogen and a hydroxyl group at the #2 carbon of the ribose sugar and that small change is enough to specify the ability of enzymes to bind to the slightly different macromolecules. So, another complication is that any protein that has DNA-binding capability would not be able to recognize the PNA.
Good point.
Great idea writing about PNAs! If I understood well, they can be used instead of shRNA to knockdown an expression of a particular gene? Compared to shRNA, how long-lasting is the effect of silencing?
PNAs are expected to last a lot longer than shRNA because they can't be degraded by any known nucleases. I would guess that once the PNA enters a cell, it'll be stuck there for long time.
Thanks for the great comment!
Thank you for the great article!
This is very interesting, because that would mean that you could potentially have "permanent" knockdown of a gene, something like with CRISPR, but better, because gene itself would retain its function.
At the moment, I'm struggling with the analyses of shRNA and CRISPR Achilles data sets, and when you compare dependency profiles for the same gene, the results are quite different, which is of course expected, since we're dealing with complete different methods (gene knockdown vs. gene knockout).
I would be very happy to see in the future some Achilles PNAs dependencies project :)
Definitely. It's possible that PNA might render some of the other silencing/knockout methods obsolete if it becomes easy to synthesize.
This was a very interesting read, @tking77798! I had no idea about how PNAs could be used to silence genes, so it was very cool to learn about this. It will be exciting to see how the future plays out for this technology, because it seems really promising if we are able to get the cost down to a decent level.
Glad you liked it!
Good article, however you mentioned that pricing has been an issue for antimicrobial PNA's; so my question is what are the efforts been made presently to break into mass production of PNA's.
Good question, and one I don't fully know the answer to. Some companies offer PNAs for purchase right now (like https://www.pnabio.com) and I assume they're working on improving their production efficiency. However, since they're part of industry, they probably keep their work secret.
Thank you
Great article! I never heard of PNA before but I'm quite convinced I will again.
Yeah! It's prevalence in research is increasing so I expect it'll become more and more popular over time. Especially as genomic data becomes more readily available. The main thing keeping it from becoming mainstream is synthesis costs at this point I think.
Yet!
I often worry there's nothing microbes can't do with a little bit of mutation!
But what you're describing sounds like a quite valuable addition to our arsenal against drug-resistant bacteria. Is it possible we've finally found the way to stop them? Very interesting.
You're definitely right that bacteria can evolve to do almost anything so PNA degradation/interaction isn't out of the question once we start using PNAs to kill them.
This is amazing, science is truly wonderful. PNA and DNA are really similar even the three hydrogen bonds and the Two hydrogen bonds in dna that holds guanine to cytosine and thymine to adenine respectively is just the same in the PNA structure. But there are two major difference I observed, PNA lack the five carbon ribose sugar and the negatively charged phosphate group. The absence of this two structure which are the two out of the three structure that make up a nucleotide makes PNA to be a partial nucleotide. It's specificity also makes it act like enzymes in that enzymes are specific in their actions.
That's right
Chic article. I learned a lot of interesting and cognitive. I'm screwed up with you, I'll be glad to reciprocal subscription))
Very interesting the article, once more extensive vision of how researchers can develop these composite of enhancing people's lives.