Operation of HDR equipment in brachytherapy.
Hello community from steemit, in this opportunity I want to inform you about the operation of HDR (High Dose Radiation) equipment used in the field of brachytherapy based on an Iridium-192 source. Which allows the treatment time to be minutes compared to the treatment of low dose radiation (LDR) that can be hours, in such a way that the patient feels comfortable when receiving treatment in vagina, prostate, lung, head and neck etc.
The HDR Unit.
El equipo de carga diferida que se muestra en la Figura 1, permite trabajar con fuentes radiactivas de forma remota utilizando computadoras, para garantizar la protección al Trabajador Expuesto Ocupacionalmente (TOE) por la alta tasa de dosis que excede los 12 Gy / h.
A deferred load HDR unit contains a single high-activity source (~ 10 Ci or 370 GBq) [1]. Although cobalt-60 and cesium-137 have been used in the past, these are located in a half-dose rate, whereas 192-Ir is the radioisotope normally used for HDR.
The source of iridium-192 used in HDR is a linear source welded to the end of a wire that allows to flexibly carry out the displacement of the source. The dimensions of the source vary between 0.3 and 0.6 mm in diameter and between 3.5 and 10 mm in length, depending on the HDR model. According to the regulations of the Nuclear Regulatory Commission [2], the leakage radiation outside the unit must not exceed 1 mR / h at a distance of 10 cm from the nearest accessible surface surrounding the shield with the source in the safe position.
In relation to the aforementioned, the Venezuelan Standard COVENIN 218-2 establishes that in the Brachytherapy equipment by remote deferred load the leakage radiation from the containers of the sources must be fulfilled.
In the containers that are installed inside the treatment room:
• The KERMA rate in air at any point 5 cm from the surface of the container, measured over an area not exceeding 10 cm², must not exceed 0.1 mGy / h.
• The KERMA rate in air at any point 100 cm from the surface of the container, measured over an area not exceeding 100 cm², must not exceed 0.01 mGy / h. In the containers that are located outside the treatment room.
• The KERMA rate in air at any point 100 cm from the surface of the container, measured over an area not exceeding 100 cm², must not exceed 1 μGy / h.
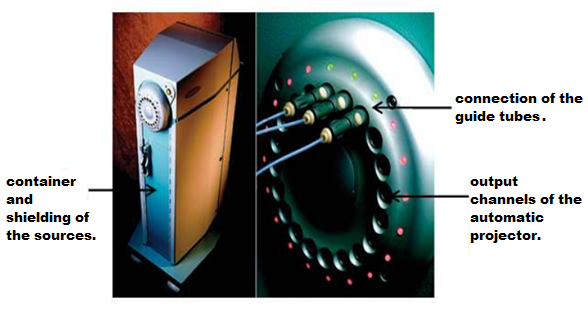
Figure 1. Deferred charge HDR Brachytherapy equipment. [3]
Radioactive sources.
Therefore, Radio has been used more frequently in Brachytherapy, which is no longer used in its long half-life, which prevents its proper protection over time and because it decays in the to highly toxic and harmful gas. However, artificial radioisotopes have special advantages in some situations, due to their γ-ray energy, the flexibility of the source, the size of the source and the half-life. Table I lists the most commonly used sources for Brachytherapy with its relevant physical properties.
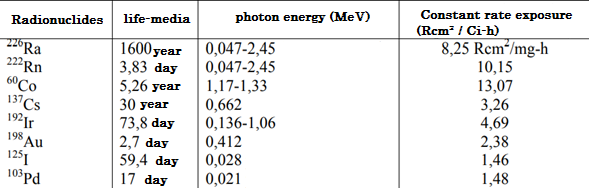
Iridium-192.
Iridium-192 has been used in Brachytherapy since 1958, initially as seeds by Ulrich Henske and then since the early 60's as wires forming the basis of the Paris system developed by Bernard Pierquin, Daniel Chassagne, Andree Dutreix and collaborators [4 ] Iridium-192 is a radionuclide with a high specific activity (or activity per unit mass), which means that a very small source can provide very high dose rates (HDR), which is essential for HDR applications and it is the most used radionuclide in HDR Brachytherapy, shown in Figure 2.
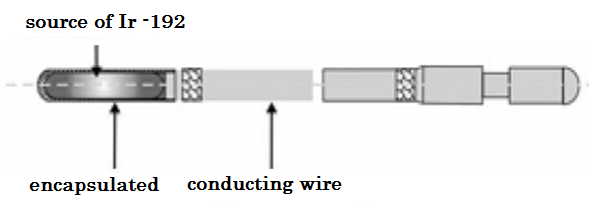
Figure 2. Iridium-192 source specifications. [5]
Table II describes the main characteristics of iridium-192, where it shows the half-life, the compound to be generated, the energy it emits, the mass of it and the energy to disintegrate.
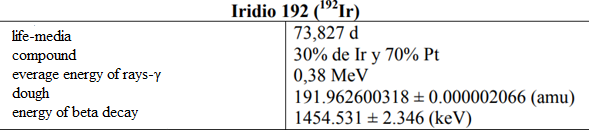
Iridium-192 is produced artificially in a nuclear reactor when iridium-192 absorbs a neutron by means of the reaction (ɳ, ɣ). This decays by electron capture to osmium-192 and by transitions β- (95.4%) to platinum-192, followed by gamma transitions and X-rays of layers K and L, as shown in Figure 3 and 4.
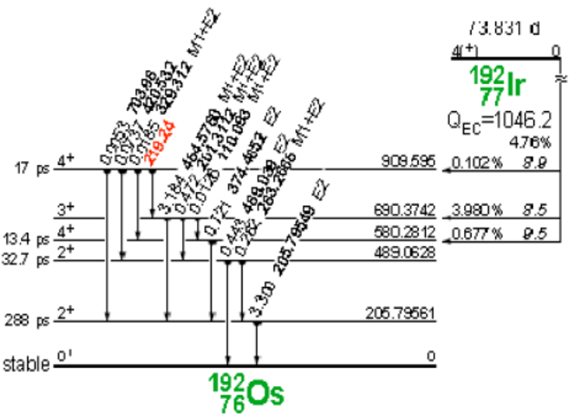
Figure 3. Decay of iridium-192 to osmium-192.
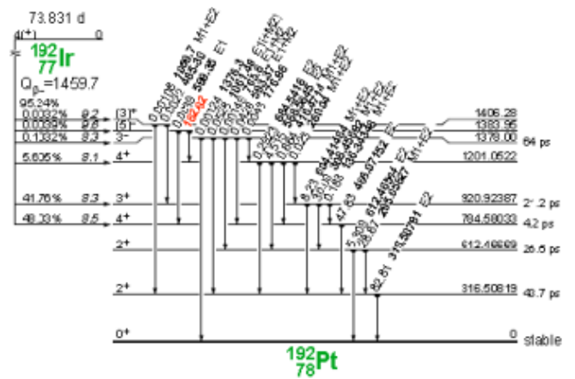
Figure 4. Iridium-192 decay to platinum-192.
REFERENCE.
[1] Khan, and Faiz, M. (2010). The physics of radiotherapy. Minneapolis Minnesota.
[2] Nuclear Regulatory Commission. (1993) Information required for the remote afterload license. Washington DC: Government Printing Office.
[3] Díaz, E., and Sánchez, M. (2008). Cancer treatments with radioisotopes: Radiotherapy. Nuclear Technology, 30.
[4] Gerbaulet, J., Pötter, R., Mazeron, J., Meertens, H., and Van Limbergen, E. (2008). The GEC ESTRO brachytherapy manual. ESTRO ISBN.
[5] Flyn, A. (2005). Isotopes and brachytherapy administration systems.
[6] JAEA. (2011). Nuclear data center. (Tokai-mura, Naka-gun, Ibaraki-ken, 319-1195, Japan) Retrieved on 21/10/2011, from http://wwwndc.jaea.go.jp/CN10/