Control of Gene Expression Part II
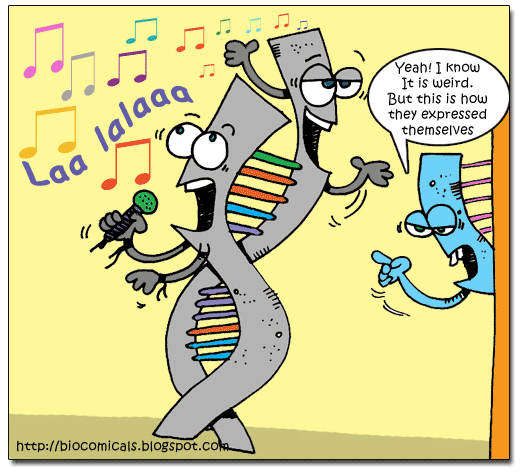
Protein-DNA interaction in gene expression and the complex architecture of eukaryotic genes, emphasizes the role of multiple interacting regulatory factors.
The same basic principles apply in eukaryotic cells as we saw in prokaryotic cells, but with many added layers of complexity that correspond to additional layers of control. We will review the basic mechanisms we learned last time in Part I, adding other details of the structure of gene regulatory regions on DNA. Part II then proceeds to outline differences in the organization of prokaryote and eukaryote genomes that require additional regulatory mechanisms to come into play. Concluding with a summary of the elements that eukaryotic cells use to turn on and off the expression of their genes.
The PaJaMo experiment confirmed Leo Szilard’s hypothesis of a repressor protein.
In the late 1950s, American biochemist Arthur Pardee began working with François Jacob and Jacques Monod. The three eventually confirmed Leo Szilard’s hypothesis of negative control for the genes for lactose-metabolizing proteins in E. coli bacteria. Their experiment (usually called PaJaMo, from the last names of each scientist) was intended to separate the effects of lactose as an inducer from the effects of the repressor protein on the expression of two enzymes, ß-galactosidase and galactoside permease. In practice, they focused on ß-galactosidase. The PaJaMo experiment took advantage of the process of conjugation, in which bacteria transfer genetic material to each other through extrachromosomal pieces of DNA, called plasmids. Bacteria are normally haploid, but bacteria that receive plasmids become diploid for any genes contained on the plasmids.
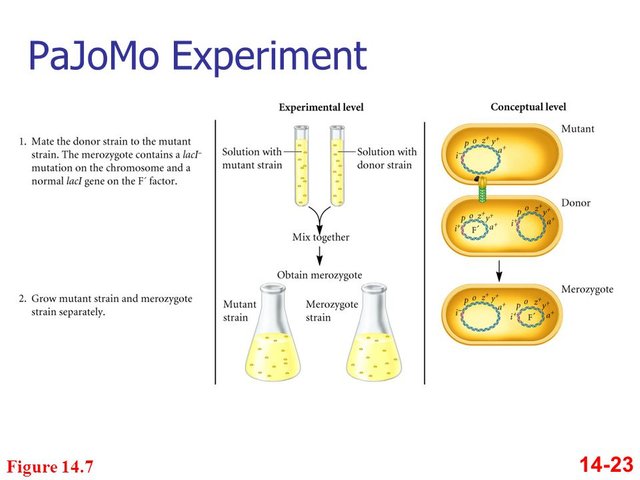
Pardee, Monod, and Jacob used bacteria with mutations in both the lacI (repressor protein) and lacZ (ß-galactosidase) genes as recipients in a conjugation experiment.
As donors, they used normal bacteria.
- Before conjugation, the recipient cells could not produce ßgalactosidase. However, because they also had defective repressor protein genes, if they received a normal ß-galactosidase gene, they would express it.
- In conjugation, the recipients received functional genes for both proteins and should then have acted like normal cells.
- What the scientists actually observed was that after conjugation, the recipient cells produced ß-galactosidase even in the absence of lactose, but only for a few hours. After those few hours, ßgalactosidase would be produced only in the presence of lactose, as is typical of a normal cell.
- The three scientists eventually realized that the two genes were transcribed at different rates. Because the lacZ gene is transcribed much more quickly than the lacI gene, it took a few hours for enough of the repressor protein to be made to shut down ßgalactosidase production.
In 1961, Jacob and Monod published a proposed summary model of gene expression.
Genetic mapping had shown that the genes for ß-galactosidase, galactoside permease, and a couple of other enzymes all occur in a single long sequence on the bacteria’s chromosome. The functional coding regions of these genes are preceded by a single promoter site. Jacob and Monod’s model proposed that there was a regulatory region, called the operator, between the promoter and the first coding region. The operator is where the repressor protein would bind to physically prevent RNA polymerase from transcribing the coding regions. Subsequent studies have shown that this is the case. Lactose binds directly to the repressor protein, changing its shape in a way that makes it unable to bind to the operator. RNA polymerase is then free to transcribe the group of lactose-metabolizing proteins.
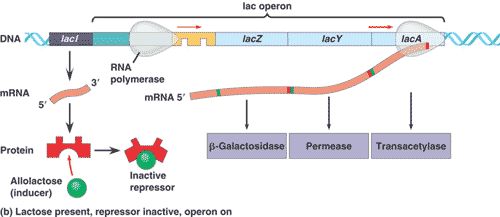
Jacob and Monod referred to the entire genetic unit the promoter, the operator, and the coding regions as an operon.
Because their operon dealt with the metabolism of lactose, it was called the lac operon. The lacI gene that codes for the repressor protein is not part of the operon but occurs nearby on the chromosome, somewhat “upstream” of the operon. We now know that E. coli has many other operons and that the binding of regulatory proteins to operators is a common control mechanism.
Prokaryotic and eukaryotic gene regulation differ in important ways, for several reasons.
Gene regulation in eukaryotes is different in many ways from gene regulation in prokaryotes, but two differences are immediately applicable.
- Prokaryotes characteristically have functionally related groups of genes that share a single promoter region and a single control mechanism. In eukaryotes, each individual gene typically has its own promoter and control regions.
- The way that regulatory proteins affect RNA polymerase is both different and much more complex in eukaryotic cells compared to prokaryotes.
Jacob and Monod’s demonstration of the way prokaryotes work, though, was an important breakthrough for both types of cells.
Among other things, it proved that some proteins exist solely to control the expression of other genes. The interactions between the repressor protein and the operator, and between the repressor and an inducer, such as lactose, are not the only influences on transcription. Another regulatory region in the lac operon helps RNA polymerase bind to the DNA strand and, thus, provides a measure of positive control over gene expression in the same way as is common in eukaryotes.
Gene regulation differs for eukaryotes and prokaryotes because, among other factors, the genomes of eukaryotes are generally much larger than those of prokaryotes.
In addition, eukaryote genomes are separated into several linear pieces (i.e., different chromosomes) and contain introns that are not transcribed. There are also large stretches of repetitive DNA whose function is not currently known. Eukaryote genomes are also tightly packaged as a complex of DNA and proteins called chromatin, whereas prokaryote DNA is unpackaged. Though they are different, gene expression in eukaryotes follows some of the general principles identified by Jacob and Monod.
Eukaryotic gene regulation is more complex than gene regulation in prokaryotes.
The most obvious difference from prokaryotes is that eukaryotic DNA is packaged in chromatin, which must somehow be unwrapped to allow RNA polymerase in. Recent research suggests that chromatin may play its own regulatory function instead of just being a supportive and protective framework. Although it is unclear how chromatin might be unpacked in a genespecific way, once the DNA is unpackaged, we are presented with the same question as in prokaryotes: How is the activity of RNA polymerase controlled to regulate gene expression? Each eukaryotic gene is associated with three kinds of regions on the DNA strand: the coding region, a promoter, and one or more regulatory regions. Certain regulator proteins must be present, and sometimes certain other proteins must be absent, for a gene to be transcribed. In addition, at least some regulatory proteins are highly selective about the DNA sequence to which they bind.
In eukaryotes, the presence or absence of regulatory proteins influences the ability of RNA polymerase to bind to a promoter.
These proteins are generally called transcription factors. A very large number of regulatory proteins may be involved in regulating transcription. Some regulatory proteins are generic and required for any gene to be transcribed; they are called basal transcription factors. A number of basal transcription factors must bind to RNA polymerase, forming a transcription initiation complex, for RNA polymerase to do its job.
Other transcription factors affect the transcription of only one or a few genes; these are called regulatory transcription factors.
Because these interact with the initiation complex, transcription involves not only protein-DNA interactions but also protein-protein interactions. Regulatory regions are normally adjacent to or even part of the promoter, but in eukaryotes, they might be very far away—up to 20,000 base pairs distant—from the genes they affect. Distant regulatory proteins are called enhancers because they seemed to enhance, rather than directly affect, transcription. It now appears that the DNA strand will actually bend back on itself, bringing enhancers into proximity to the genes they control.
As in prokaryotes, regulatory proteins can have either a positive or negative effect on transcription; however, in eukaryotes, all regulation involves the binding of RNA polymerase and the initiation complex, rather than simple physical barriers.
Would the “Pa-Ja-Mo” experiment have supported Szilard’s hypothesis of negative control in the lac operon if the lacZ gene and the lacI gene were transcribed at the same rate? Why or why not?
Having many regulatory proteins controlling the transcription of a gene seems unnecessarily complex, just like using many keys to start a single car. What are some possible advantages of this added complexity?
Image Credits -
http://3.bp.blogspot.com/-CbPtclMayF0/Tc55-LTxghI/AAAAAAAADCo/x7Soyvz3JHs/s1600/GeneExpression.gif
http://images.slideplayer.com/10/2810804/slides/slide_20.jpg
http://biologyboom.com/wp-content/uploads/2014/07/lacoperon.gif
http://schoolbag.info/biology/living/living.files/image350.jpg
https://upload.wikimedia.org/wikipedia/commons/thumb/8/80/Transcription_Factors.svg/1200px-Transcription_Factors.svg.png
Bird [Giphy](https://giphy.com/gifs/blue-5lDik5jPpRw2Y)
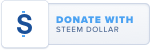
BTC: 1GfXFX1o1uUkC4HuGuKUPty6wdu6oi8X1t
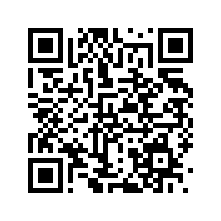
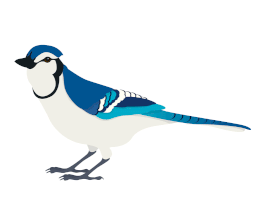